On The Clinical Applicability Of Superparamagnetic Nanoparticle Tomography
- JYP Admin
- Sep 5, 2023
- 5 min read
Updated: Oct 8, 2023
Author: Dhruv Hegde
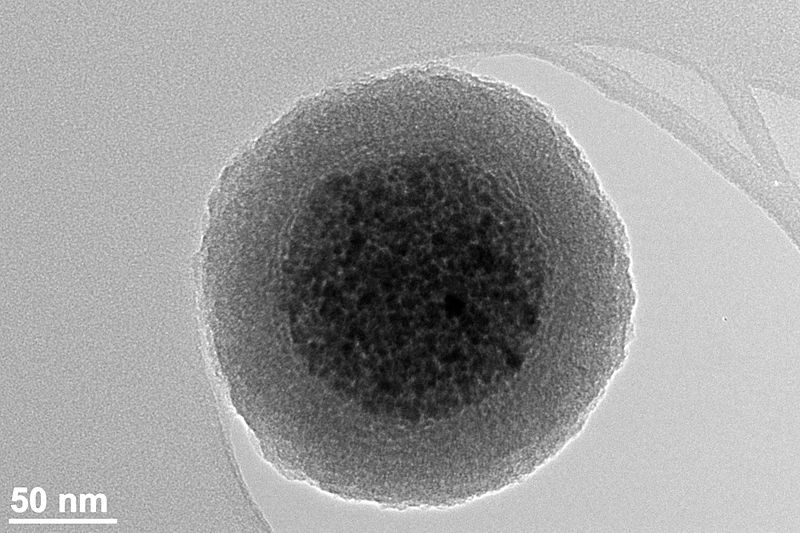
[Source: Marko Petek]
Abstract
This article seeks to analytically examine the current performance of Magnetic Particle Imaging, the form of Superparamagnetic Nanoparticle Tomography that is currently being developed for clinical application. Features of Magnetic Particle Imaging in relation to similar counterparts, including Computed Tomography and Positron Emission Tomography, are discussed through a computational perspective; this article addresses the functioning and efficiency of various metal nanoparticles and the operation of paramagnetic particles at a quantum level with respect to biophysics. Given that Magnetic Particle Imaging is yet to be clinically applied on macroscopic organisms to a great degree, the clinical applicability and future development needed in the field of Magnetic Particle Imaging are also discussed. Through the use of alternative superparamagnetic nanoparticles and imaging applications, the field could be ameliorated to provide more positive results to indicate a greater probability of involved implementation.
Background
Tomographic techniques have been a prevalent force in contemporary biomedicine and biophysics, with it not only helping to advance modern quantum information and plasma physics, but also creating a significant force in radiology. Tomography is the physical technique of utilizing an external wave to capture a cross-sectional image of a standard entity; in this way, tomographic sections are projections of layers within a certain object. Computer Tomography, a common medical imaging technique, utilizes standing X-rays and measures the attenuations of these very wavelengths within the human tissue, which can then be measured and mapped accordingly to create a simulated projection of a human tissue layer. The process for a Computed Tomographic projection is replicated in many additional forms, so it is necessary to outline the basic developments of the process. Within the machine that is utilized to fabricate the final project, there is a circular opening that allows for the patient to be directly inserted within. From here, the internal cylinder wall, where the X-ray emitter is located, revolves at one revolution per second; the reason this is done is to create a uniform distribution of X-rays to be sampled. Following this, X-rays are concentrated on the portion of the body that needs to be mapped. Once this occurs, sensors that line the internal wall of the CT machine look for absorbance or reflection patterns and fabricate a snapshot of the given later of the body. Originally introduced by Hounsfield and Cormack, computer tomography became a practice technique in radiology and offered a promising base for astrophysical applications as well.
Positron Emission Tomography is a form of functional imaging and projection measuring that is a prevalent technique in biophysics and medicine. Rather than measuring the returning wavelength patterns of particle emissions within the body, the Positron Emission Tomography technique utilizes radioisotope tracers, such as Fluorodeoxyglucose, for the purpose of measuring changes in blood flow and metabolic activities. The base for the Positron Emission Tomography comes from the emission of a positron, or an anti-electron, from the nucleus of the radiative agent that is injected. The positron, colliding and interacting with the atoms in the tissue causes electron interaction that produces multiple photons to be emitted in the form of energy. These photons provide the ability for the scanner to detect them and produce the image based on the mapping from their approaching location.
The radioactive agents are not eminently radioactive or harmful to the human body, but have been known to cause minor repercussions to patient health if used repeatedly in testing; generally, the radioactive material is internally processed and excreted naturally following a period of several days, leaving no known long-term health ramifications to the patients. However, more concerning than the minimal health effects is the computed inaccuracy that PET could have as a result of chemical displacements within the body, causing severe limitations towards its use. For example, PET scans have produced many false positive results when faced with lesions or tumors, which is the preeminent limitation for the use of PET scans on serious diseases.
History of Magnetic Particle Imaging
Magnetic Particle Imaging is a relatively contemporary innovation in the field of computational biophysics that operates in a way similar to Positron Emission Tomography in that it involves tracers and emission gatherers. Invented in 2001 by researchers in Hamburg, Magnetic Particle Imaging is a non-invasive form of detection that utilizes various chemical nanoparticles as tracers. These nanoparticle tracers, which have an atomic diameter of merely dozens of angstroms, have been refined to the nanometer. Based on the current research on lymph node biopsy, 32 nm Ferumoxytol and 111nm Ferumoxide are the most implementable tracers in biophysical applications. Magnetic Particle Imaging is done by invoking a changing magnetic field from outside to the body to observe the behavior of the nanoparticle as it travers the human body; based on Faraday’s law of induction, an electrical field is invoked and causes a change in the magnetic flux. Once this change in the magnetic flux occurs, the nanoparticles respond to this change in the magnetic field by reorienting its spin to an opposing vertical position.
Magnetic Particle Imaging, although solid in conception, lacks strength in design constructs within a confined area, and, by extension, budget. Within the basic construct, an MPI imager utilizes a strong magnetic field gradient that is produced as a direct result of the imaging volume by the main magnet; within this gradient, periods of high and low magnetic fields are fluctuated and rotated. When the weaker magnetic fields, known as field-free regions, cross paths with the superparamagnetic particle, the flip that was discussed previously occurs instantaneously. Through the controlled rotation, this can be measured, and so the field-free regions are constantly rotated to cover large portions of imaging volume to map out the quantum properties of the superparamagnetic particles and observe their relative location.
Applicability of Magnetic Particle Imaging
Given the relative position of Magnetic Particle Imaging as a technological aid to medicine, the experimental uses for Magnetic Particle Imaging lie adjacent to similar established technologies; however, the applicability at its current state remains dire given its lack of testing on human patients or organisms of such high mass. In computational models, and imaging platforms such as vivo, MPI continues to pave the way for a biologically safe and relatively cost-effective substance that can be reliably produced and stored at clinical facilities. The noninvasive signaling techniques that are used in MPI also signal its use in deep tissue measurements with a high degree of temporal clarity and resolution; cancer imaging can be done in line with the biological tracking of the provided combatants. Alongside this application, it has also been clinically tested and certified for cell-based therapy within oncology. MPI is also not limited to somatic cells and has also been used to track neural and mesenchymal stem cell growth. As the domain of Medicinal Physics continues to expand, the near future shall unravel new research that can ameliorate the applicability of such a technology and allow for it to become a feasible and universal system.
Bibliography
Ajayi, Tolulope O., Sitong Liu, Chelsea Rosen, Carlos M. Rinaldi-Ramos, Kyle D. Allen, and Blanka Sharma. 2023. “Application of Magnetic Particle Imaging to Evaluate Nanoparticle Fate in Rodent Joints.” Journal of Controlled Release 356 (April): 347–59. https://doi.org/10.1016/j.jconrel.2023.02.038
Han, Xiao, Yang Li, Weifeng Liu, Xiaojun Chen, Zeyu Song, Xiaolin Wang, Yulin Deng, Xiaoying Tang, and Zhenqi Jiang. 2020. “The Applications of Magnetic Particle Imaging: From Cell to Body.” Diagnostics 10 (10): 800. https://doi.org/10.3390/diagnostics10100800
“Magnetic Iron Oxide Nanoparticles for Magnetic Particle Imaging | MIT Technology Licensing Office.” n.d. Tlo.mit.edu. Accessed July 26, 2023. https://tlo.mit.edu/technologies/magnetic-iron-oxide-nanoparticles-magnetic-particle-imaging
Paysen, Hendrik, Norbert Loewa, Anke Stach, James Wells, Olaf Kosch, Shailey Twamley, Marcus R. Makowski, Tobias Schaeffter, Antje Ludwig, and Frank Wiekhorst. 2020. “Cellular Uptake of Magnetic Nanoparticles Imaged and Quantified by Magnetic Particle Imaging.” Scientific Reports 10 (1): 1922. https://doi.org/10.1038/s41598-020-58853-3
“Single-Sided Magnetic Particle Imaging Device with Field-Free-Line Geometry for in Vivo Imaging Applications.” n.d. Scholar.google.com. Accessed July 26, 2023. https://scholar.google.com/citations?view_op=view_citation&hl=en&user=EvSdqHYAAAAJ&citation_for_view=EvSdqHYAAAAJ:YFjsv_pBGBYC
Comentários